The James Webb Space Telescope has just produced yet another stunning image: the planet Neptune, glittering like a mirrorball, as its rings and moons dance around it. Humanity’s newest, best and most expensive telescope has dazzled us again and again in its first few months of operation, and will continue to do so for another couple of decades. But perhaps the most astonishing image it has produced so far is one of the least dramatic: the first of its photographs ever released. I suspect many of us still do not understand how astonishing that image is, and why. I will attempt to explain both.
[ Neptune and its rings shown in new light by Webb space telescopeOpens in new window ]
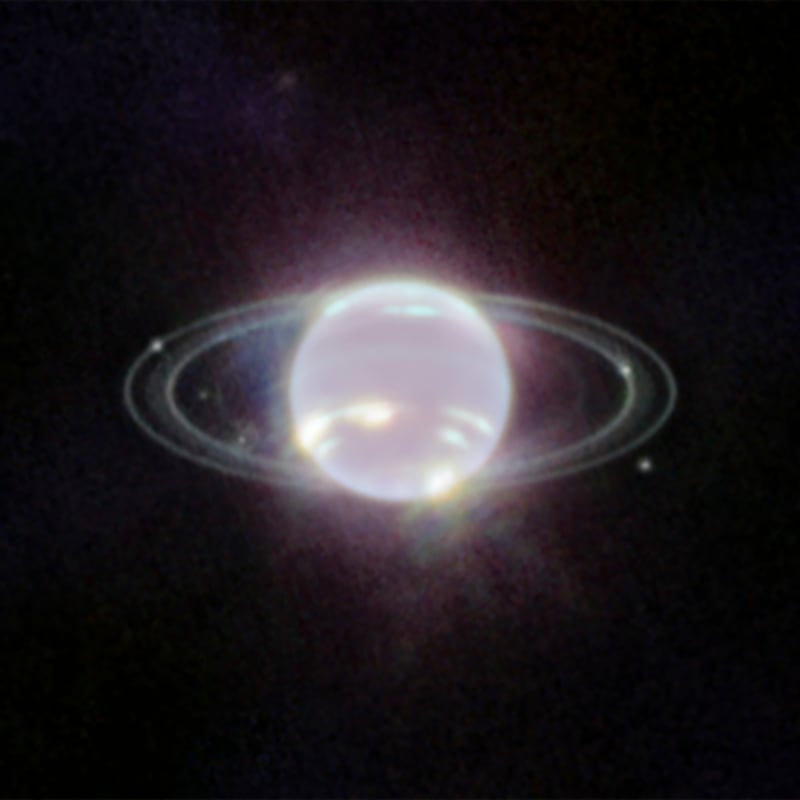
How to see light from the dawn of time
I’ve spent the past decade researching a book that redescribes the universe. Yes, all of it. (Yes, my head hurts.)
And something I’ve slowly realised is that most non-scientists don’t really understand this stuff, even if they think they do.
Another thing I’ve realised is that most scientists don’t remotely understand how little most non-scientists understand it, so scientists’ explanations of these things are largely useless.
‘The minute I sat down on the train, I knew I’d been scammed’: Are the Irish susceptible to con artists?
From Billie Eilish to Electric Picnic: 85 unmissable concerts and festivals in Ireland in 2025
‘That would have never happened in Ireland,’ my boyfriend said after my trip to Australian A&E
Depression came down on me like a terrible cloud. I thought it would never lift, but it did
That’s a real pity, because if you don’t understand what red shift is, or the astonishing technical difficulty of observing infrared light (which is basically heat) from the surface of a warm planet like Earth, then you can’t really appreciate a picture such as the very first photo the James Webb telescope revealed, of countless galaxies, getting tinier and redder and older as they stretched back closer and closer to the big bang. As my parents said, when we talked about it afterwards, “We saw them, but we don’t know what we’re looking at.”
But if you do understand a few things about light, and space, and time, that picture will blow your goddam mind.
Sending back pictures of the early universe ... Wait, what?
That picture is a picture of the early universe. Scientists keep saying that — they said it again and again on the day they revealed the image — but they are so familiar with the idea that they rarely unpack it fully. Sending back pictures of the early universe. That’s an achievement so stunning, so crucial to appreciating that first James Webb photo, and so hard to fully take in, that we should definitely unpack it a lot more.
Borne ceaselessly into the past
Telescopes capture light. Light travels in little packets called photons that behave awfully like waves. Some of those waves are a lot longer than others, but they all travel at exactly the same speed. Okay, now forget all that. Here’s the stuff that matters…
Because nothing can travel faster than the speed of light — not even, um, light — all telescopes are always looking into the past.
If you’re just looking around our neighbourhood — ie, all the places humans have ever been — that’s not a big deal. Light travels 300,000km a second, and Earth is only 40,000km around, so you can see anything on Earth pretty much in real time (even if you have to bounce the signal off a satellite).
Even light from the moon, about 385,000km away, gets here in not much more than a second.
But light from our sun takes eight minutes to get here.
Light from the next-nearest star (Proxima Centauri — absolutely rubbish little star, unfortunately) will take four years to get here.
Our galaxy is a complex, swirling spiral containing a hundred thousand million stars, and light from the far side of it takes 100,000 years to get here. (Put another way, our galaxy is 1,000,000,000,000,000,000km across: any light reaching us from the far side of it started out before the last ice age.)

Light from the next nearest spiral galaxy, Andromeda, started its journey shortly after our ancestors in Africa started using stone tools, 2.5 million years ago.
And the edge of the visible universe is roughly 20,000 times farther away than that.
So light from the early universe is still arriving on Earth today, after an incredibly long journey.
But looking at things that are farther away isn’t simply a matter of building a bigger telescope to magnify and see them. Visible light from the early universe — light in the frequency range that human eyes can detect — is no longer visible to human eyes by the time it arrives here. Why?
Because, as you look farther and farther away (and so farther back in time), something else starts to become significant: all the light you see is not just dimmer but also redder, as its wavelength stretches out and gets longer. That is, there is not just less light, but that light has less energy.
To understand what’s going on here, it might be useful to have a quick aside on light.
A quick aside on light
Remember, humans can see light in an astonishingly narrow range of wavelengths — from 400 to 700 nanometres. So from just under half of a billionth of a meter to just over two-thirds of a billionth of a meter. (Why did we evolve to detect those particular wavelengths? Because that’s the frequency range in which our sun produces the most light. We are children of our particular planet, our particular sun.)
In other words, we can see the thinnest possible slice of an enormously (almost infinitely) broad potential spectrum that extends away in both directions. Light exists, to all intents and purposes, at all wavelengths. There is light millions of times shorter than the light you can see — and billions of times longer.
Light with a very short wavelength can punch right through solid matter with so much energy it’s dangerous. That is how X-rays work: light punches right into you; some of it is stopped by your bones, and the remaining light, as it exits you, is then captured to make a picture of your insides.
And if you think that is pretty hard-core behaviour for a beam of light, gamma rays are a thousand times shorter, and thus punchier, than that. In fact, gamma rays can be shorter than a trillionth of a metre in length: they are so short in wavelength, so compact, so punchy, so small, that they pass between the atoms of detectors. (To detect gamma rays you need to use a big, solid block of some densely packed crystal, and hope they hit something on the way through.)
Indeed, a gamma ray (the Dracula of photons) is not reflected by a mirror, because the gamma ray is so small that it sees the mirror not as a flat surface but as lots of atoms, far apart. And so the gamma ray just whizzes through the gaps.
Put another way, it takes a thousand visible light waves to cross the width of a single human hair. But you could fit a million gamma waves into each of those thousand visible light waves.
By contrast, light with a very long wavelength, like the light we call radio waves, is so low in energy it’s completely harmless. The radio waves we listen to on an FM radio are a couple of metres long; the radio waves on a crackly old AM radio can be hundreds of metres long.
The thing is, we’ve known since 1929 that the farther back you look the more weirdly the light behaves. Light that should be visible to our eyes, for instance — the light of stars like our own sun — changes as the source gets farther away. Its wavelengths get lower, its energy falls and it becomes harder and harder to see, until it drops below the frequency/energy range our eyes can detect at all.
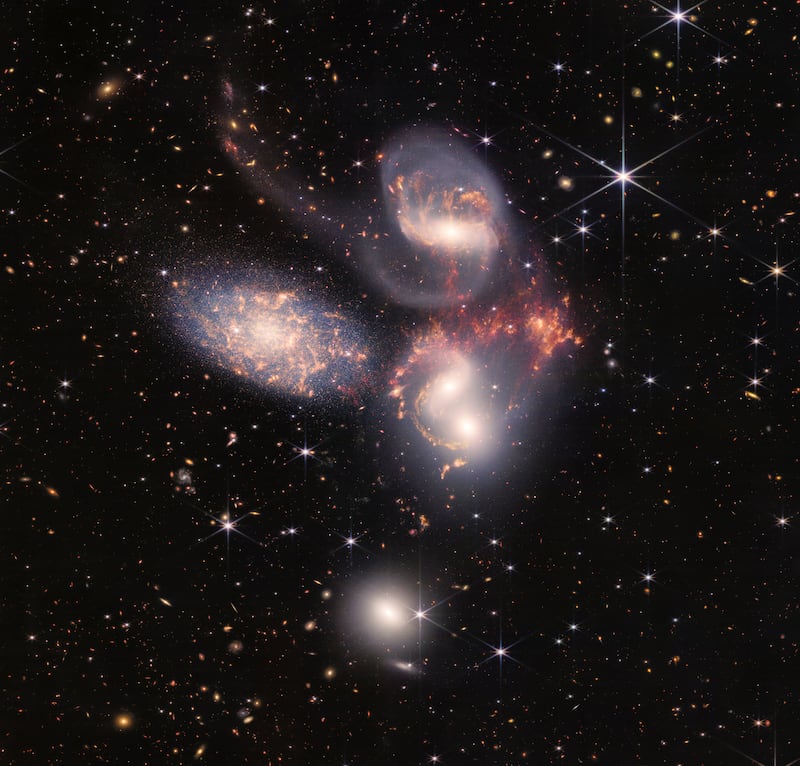
Oh, that’s why it’s called red shift!
Yes, the process is called red shift because it shifts visible light towards the red end of the spectrum.
For example, the wavelength of red light is about twice as long as the wavelength of blue light, so stretch blue light waves to twice their original length and they will look red. (Indeed, they will now be red.) Stretch red light and it will become infrared: the wavelengths are now too long for our eyes to detect, so we won’t be able to see that light at all.
To understand why this happens, you need to understand what’s been happening to space-time and thus to the light travelling through it, since the big bang.
The big bang was not a normal explosion
There is a very human tendency to think of the big bang as an explosion, but that’s not quite right. An ordinary explosion expands into the surrounding space-time — if a bomb goes off in a street, the debris is scattered all over the street. But in the big bang, space-time itself is expanding, which stretches everything in the universe farther and farther apart as time goes on. It’s more as though the street itself were getting longer, and the gaps between the buildings growing larger.
To imagine how this is happening to the entire universe, draw some galaxies on a deflated balloon. Now blow it up and watch the space between the galaxies get bigger even though the ink (=matter) of the galaxies isn’t moving relative to the rubber surface (=space-time) of the balloon. The expansion of space-time is a bit like that.
That doesn’t mean everything is receding from everything else. Things that are close enough to be bound together by gravity will remain together — you will stay on Earth’s surface; Earth will continue to orbit the sun; the stars in our galaxy will continue to rotate together, and the local cluster of galaxies will stay in touch with each other. (Indeed, our neighbouring spiral galaxy, Andromeda, is so gravitationally attracted to our own Milky Way galaxy that we are due to collide in about another four or five billion years.)
But, as time goes by, our cluster will continue to recede from all the other gravitationally bound galaxy clusters, as they all recede from each other, until (eventually, after many tens of billions of years) nothing in the universe will be reachable by us, even at the speed of light, except our local cluster.
So how much has the expansion of space-time since the since the big bang stretched light?
Because the universe has been expanding for the 13.8 billion years since the big bang, the light waves travelling through that space-time have been stretched along with it. Stretched a lot.
Light emitted very soon after the big bang would have been stretched out to dozens of times its original length by the time it finally gets to Earth.
That means visible light from the early universe has been stretched so much that it has dropped deep into the infrared by the time it reaches us, and we see it. So we don’t see it (or at least we never have, until now). Because infrared light is basically heat, the kind of light everything warm gives off.
This is a huge problem for telescopes, because everything on Earth, including our telescopes, and including the air itself, is warmer than the incredibly faint, extremely red-shifted light of the early universe — and our warmth drowns out its faint signal. Imagine using infrared goggles that are on fire to look through a nearby burning forest for a glimpse of a distant ice cube.
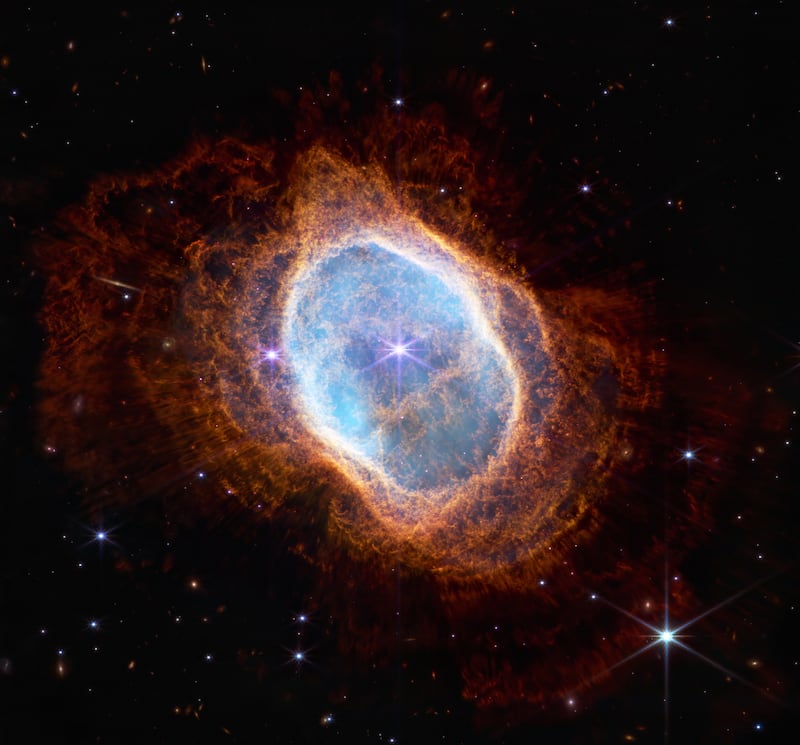
Introducing our hero, the James Webb Space Telescope
So to see that far across the universe, and thus that far into the past, the James Webb Space Telescope (which is designed specifically to see that infrared light) has had to travel more than 1.5 million kilometres from hot, noisy Earth; has blocked the hot, noisy sun, with a heat shield five layers thick and the size of a tennis court; and has refrigerated its most sensitive infrared detector down to minus 266 degrees, which is only 7 degrees above absolute zero.
It’s the astonishing, triumphant result of deep collaboration between intellectual thought and physical craftsmanship across multiple decades: the technological equivalent of Chartres Cathedral. When I complain about the limits of reductionist materialism, as I often do, I am complaining about the limits — and about our failure to do other stuff that needs doing beyond those limits. I am not complaining about the approach itself, because, holy crap, modern reductionist science can launch a cathedral full of technological miracles into space.
The miracles continue once that data is beamed back to Earth. You still can’t see infrared light with your human eyes, so technicians must choose how to render that information, in colour, as photographs. They must make aesthetic decisions about the shades of blue and red and orange that will represent these captured wavelengths that no human eye can ever witness unaided.
And so in that first James Webb photograph we are at last seeing light that started out well over 13 billion years ago, light that has been stretched to invisibility on its voyage, finally arriving from an early universe we’ve never seen before, and boosted back up into visibility by both technology and art.
We are blinking our eyes in astonishment at the light from the dawn of time.
Julian Gough is the author of the science-fiction novel Connect (Picador), the Rabbit & Bear books with Jim Field, and the ending to the computer game Minecraft. He is currently redescribing the universe at The Egg and the Rock